Quantum Computing Breakthrough: Diamond Qubits Achieve Unprecedented Precision
Quantum Computing Breakthrough: Diamond Qubits Achieve Unprecedented Precision
April 28, 2025
Groundbreaking Progress in Quantum Gate Fidelity
In a landmark advancement for quantum computing, scientists at QuTech—a collaboration between Delft University of Technology and the Netherlands Organisation for Applied Scientific Research (TNO)—have shattered previous records by developing ultra-precise quantum gates on a diamond-based chip. Their system achieves error rates as low as 0.001%, a critical milestone that brings fault-tolerant quantum computing closer to reality.
This breakthrough, published in Physical Review Applied, demonstrates that diamond spin qubits can perform high-fidelity quantum operations, overcoming one of the field’s most persistent challenges: maintaining precision across long sequences of computations.
Why Quantum Gates Must Be Nearly Perfect
Quantum computers rely on quantum gates—the fundamental operations that manipulate qubits—to perform calculations. Unlike classical bits, qubits are highly sensitive to noise, meaning even tiny errors can cascade and derail computations. For quantum error correction (QEC) to work effectively, gate errors must remain below 0.1%, a threshold known as the "fault-tolerant limit."
Until now, most quantum platforms struggled to consistently meet this benchmark. QuTech’s diamond-based system not only surpasses it but does so with some gates operating at 0.001% error rates, making it one of the most reliable quantum technologies to date.
Diamond Qubits: A Leading Candidate for Scalable Quantum Computing
The researchers utilized nitrogen-vacancy (NV) centers in diamond—atomic-scale defects where a nitrogen atom replaces a carbon atom, trapping an extra electron. These defects act as natural qubits, offering several advantages:
1. High-Temperature Operation
Unlike superconducting qubits, which require near-absolute-zero temperatures, diamond spin qubits can function at up to 10 Kelvin, significantly reducing cooling demands.
2. Exceptional Noise Resistance
The diamond lattice naturally shields qubits from electromagnetic interference, making them more stable than competing technologies.
3. Built-in Photon Coupling
NV centers can interact with light, enabling quantum networking—a crucial feature for future distributed quantum computing.
Despite these benefits, previous attempts to build high-fidelity quantum gates with diamond qubits were hampered by material imperfections and control challenges.
How QuTech Achieved Near-Perfect Quantum Gates
The team’s success hinged on three key innovations:
1. Ultra-Pure Diamond Crystals
Natural diamonds contain carbon-13 isotopes, which introduce magnetic noise. The researchers used isotopically purified diamonds, drastically reducing decoherence.
2. Advanced Gate Design
By carefully engineering microwave and laser pulses, they minimized unwanted interactions between qubits and their environment.
3. Precision Characterization with Gate Set Tomography
Instead of traditional benchmarking, the team used gate set tomography (GST), a method that provides a complete quantum description of each gate. "GST was essential—it revealed hidden errors that standard tests miss," explains co-author Jiwon Yun.
Stress-Testing the Quantum Gates
To validate their system, the researchers ran an 800-gate quantum algorithm, far exceeding typical test sequences. The results matched theoretical predictions with near-perfect accuracy, proving the gates’ reliability.
"This level of precision means we can trust our quantum operations even in long computations," says lead author Hans Bartling.
The Next Challenge: Scaling Up
While this two-qubit demonstration is a major leap, building a practical quantum computer requires thousands—or even millions—of qubits. Key hurdles include:
Maintaining low error rates as qubit count increases
Integrating diamond qubits with photonic interconnects
Developing scalable fabrication techniques
QuTech is tackling these challenges through its collaboration with Fujitsu, combining expertise in quantum hardware, control electronics, and system architecture.
"We’re not just improving qubits—we’re rethinking the entire quantum computing stack," says Tim Taminiau, the project’s lead scientist. "The next phase will require industry partnerships to transition from lab experiments to real-world applications."
Implications for the Future of Quantum Computing
This breakthrough has far-reaching implications:
✔ Fault-Tolerant Quantum Computing – Proves diamond qubits can meet the strict error thresholds needed for QEC.
✔ Hybrid Quantum Systems – NV centers could link superconducting or trapped-ion qubits via photons.
✔ Quantum Networks – Enables long-distance entanglement for secure communication.
Conclusion: A Major Step Toward Practical Quantum Computers
QuTech’s diamond-based quantum gates represent a turning point in the quest for scalable quantum computing. While challenges remain, this work demonstrates that high-fidelity quantum operations are achievable, paving the way for error-corrected, large-scale quantum processors.
As research progresses, diamond qubits may soon compete with—or even surpass—other leading quantum technologies, bringing us closer to solving problems beyond the reach of classical supercomputers.
Reference:
H.P. Bartling et al., "Universal high-fidelity quantum gates for spin qubits in diamond," Physical Review Applied (2025).
DOI: 10.1103/PhysRevApplied.23.034052
April 28, 2025
Quantum Insights



Cracking RSA with Fewer Qubits: What Google's New Quantum Factoring Estimate Means for Cybersecurity
May 28, 2025



Quantum Arms Race: U.S. Defense Intelligence Flags Rivals’ Growing Military Use of Quantum Tech
May 27, 2025



Quantum Threats and Bitcoin: Why BlackRock’s Warning Matters for the Future of Crypto Security
May 27, 2025



Sudbury's SNOLAB Ventures into Quantum Computing Research
May 26, 2025



Lockheed Martin and IBM Pioneer Quantum-Classical Hybrid Computing for Complex Molecular Simulations
May 23, 2025



Why the Moon Matters for Quantum Computing: From Helium-3 to Off-Planet Quantum Networks
May 23, 2025



NIST Approves Three Post-Quantum Cryptography Standards: A Milestone for Digital Security
May 22, 2025



Scientists Connect Quantum Processors via Fiber Optic Cable for the First Time
May 21, 2025



Quantum Computing and Encryption Breakthroughs in 2025: A New Era of Innovation
May 21, 2025



How CISOs Can Defend Against the “Harvest Now, Decrypt Later” Threat
May 20, 2025



NVIDIA Expands Quantum and AI Ecosystem in Taiwan Through Strategic Partnerships and Supercomputing Initiatives
May 19, 2025



Quantum Annealing Breakthrough: Quantum Computer Outperforms Fastest Supercomputers
May 18, 2025



Quantum Computing's New Frontier: How the $1.4 Trillion US–UAE Investment Deal is Shaping the Industry
May 16, 2025



Quantum Computing Meets Cancer Research: A New Frontier in Drug Discovery
May 16, 2025



Quantum Industry Leaders Urge Congress to Reauthorize and Expand National Quantum Initiative
May 15, 2025



Honeywell's Quantinuum and Qatar's Al Rabban Capital Forge $1 Billion Quantum Computing Joint Venture
May 15, 2025



Advancing Quantum Machine Learning with Multi-Chip Ensemble Architectures
May 14, 2025



How will the new US-Saudi Arabia AI deal effect the Quantum Computing industry?
May 14, 2025



Saudi Arabia's $600 Billion AI Push: Amazon, Nvidia, and Global Tech Giants Lead the Charge
May 14, 2025



Quantum Computing Breakthrough: Diamond Qubits Achieve Unprecedented Precision
Apr 28, 2025



Australia’s Quantum Cryptography Roadmap: Preparing for a Post-Quantum Future
Apr 26, 2025
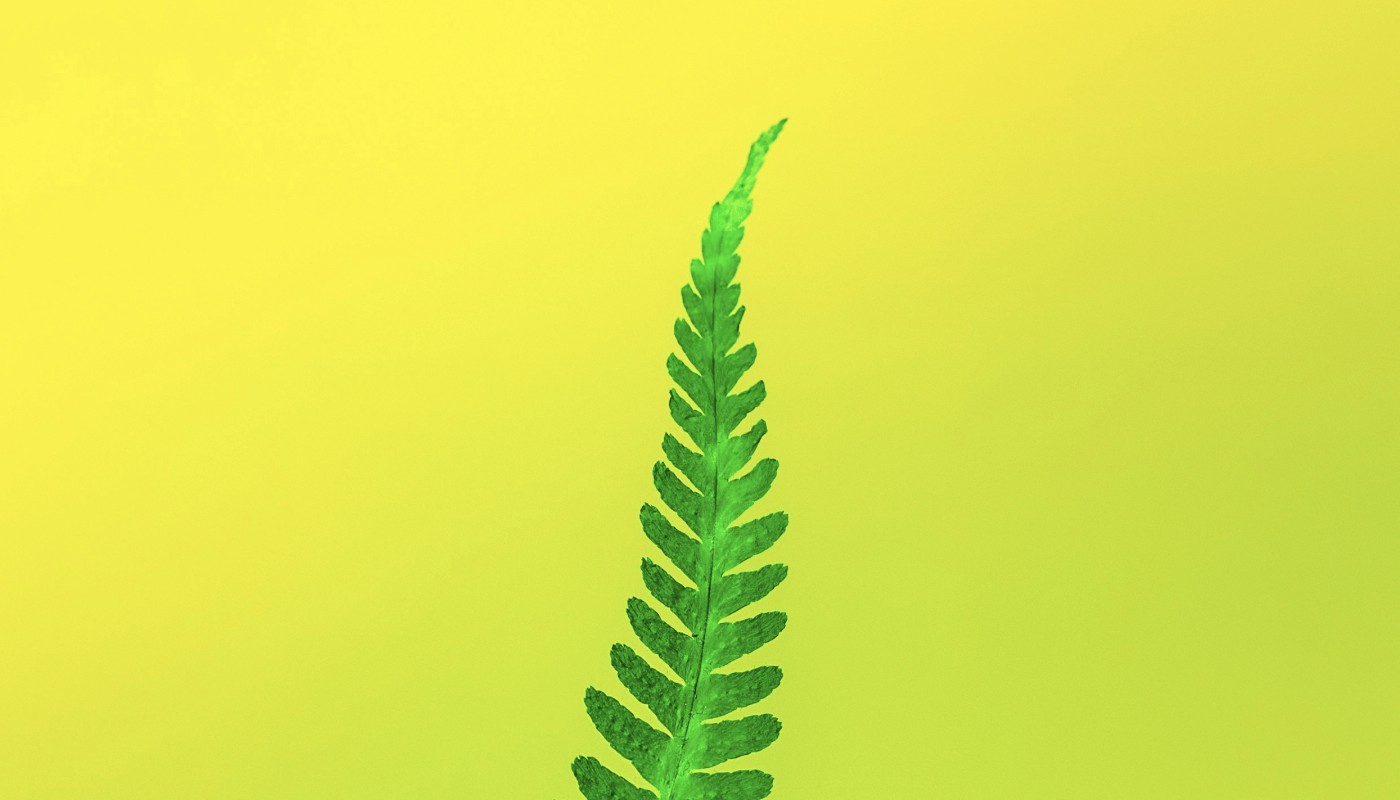
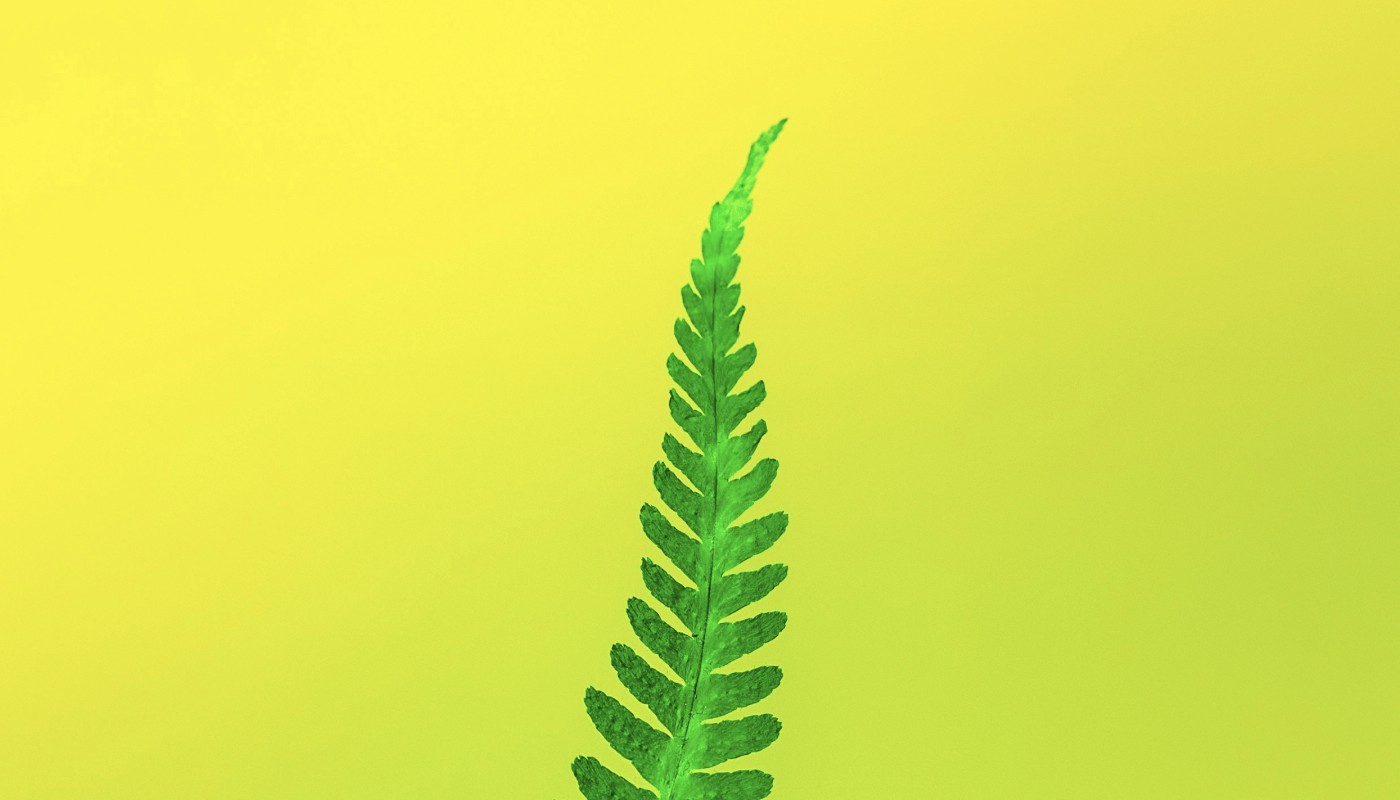
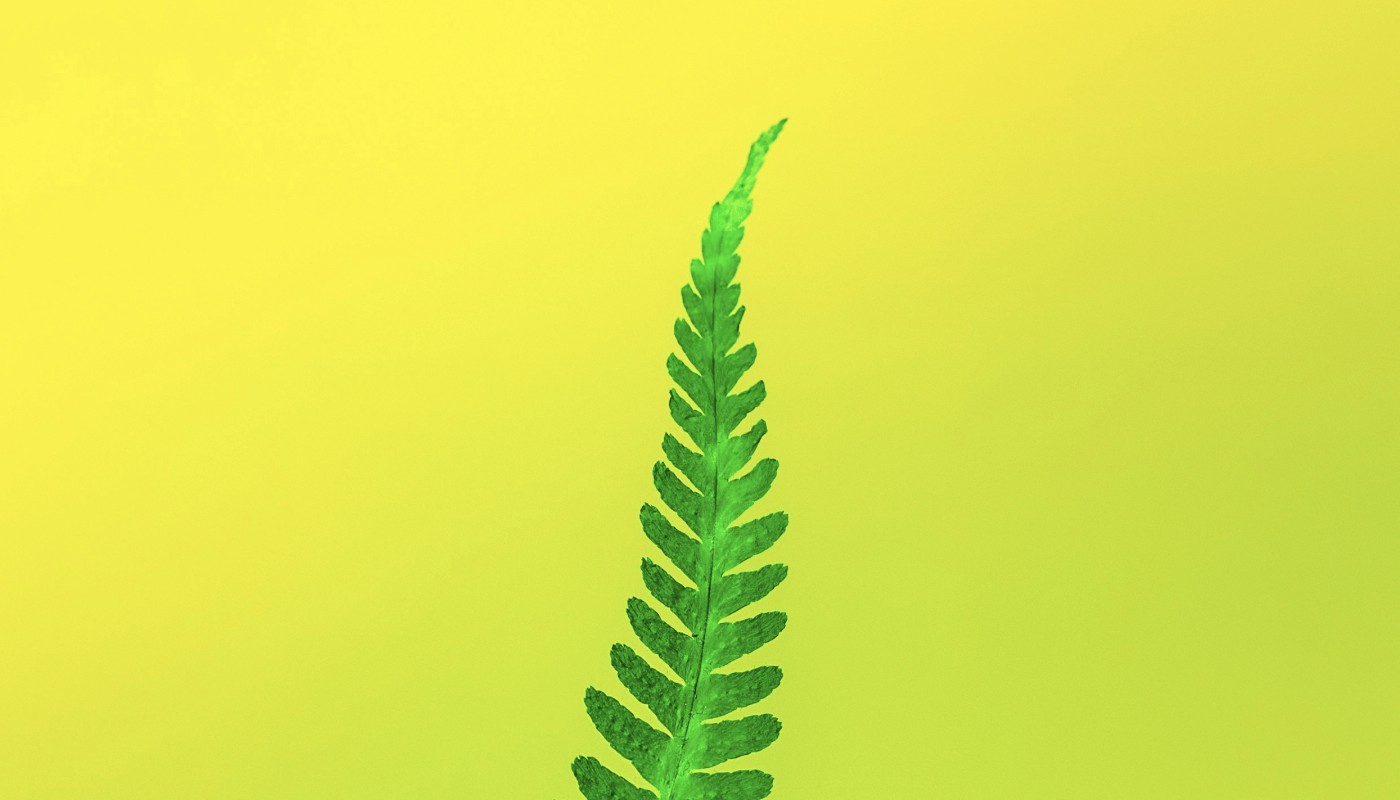
Harvest Now, Decrypt later
Apr 25, 2025
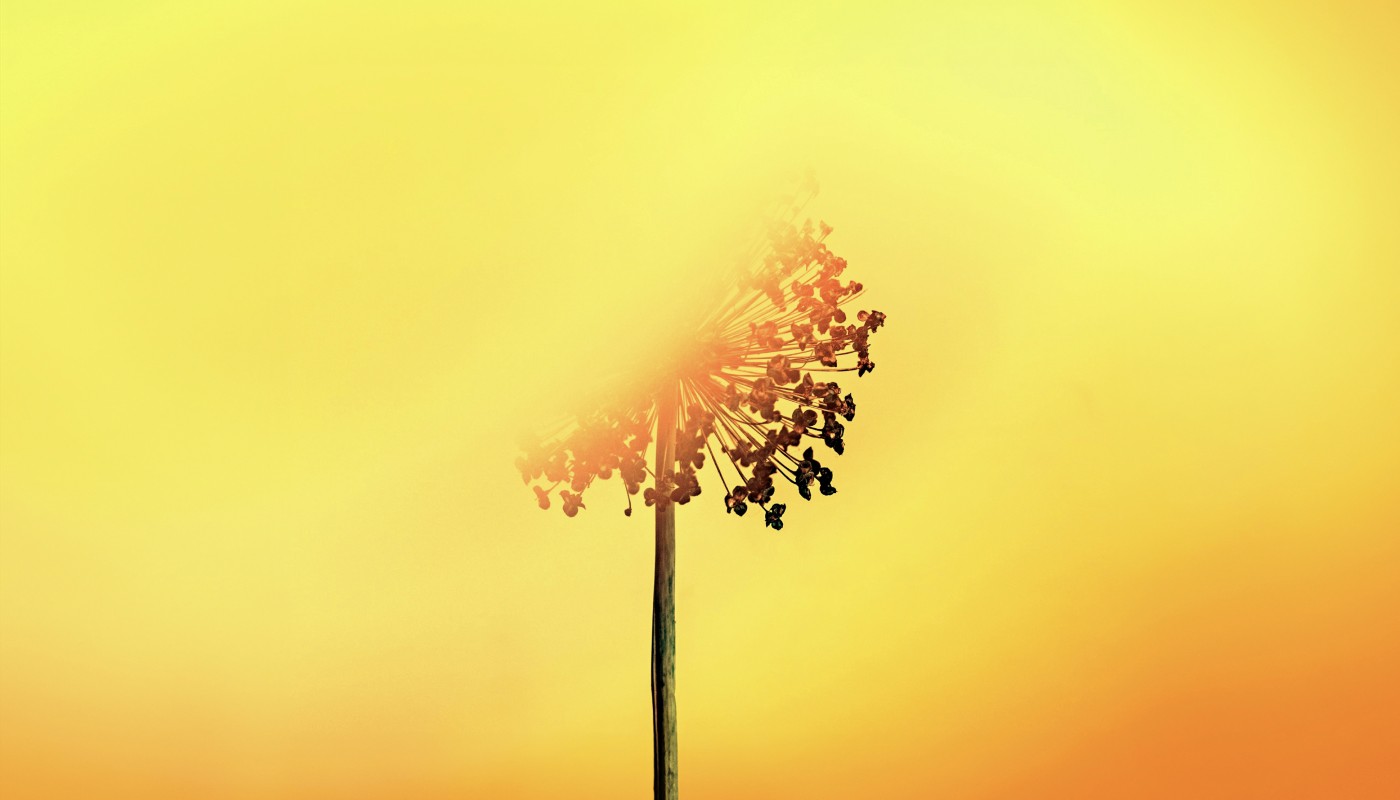
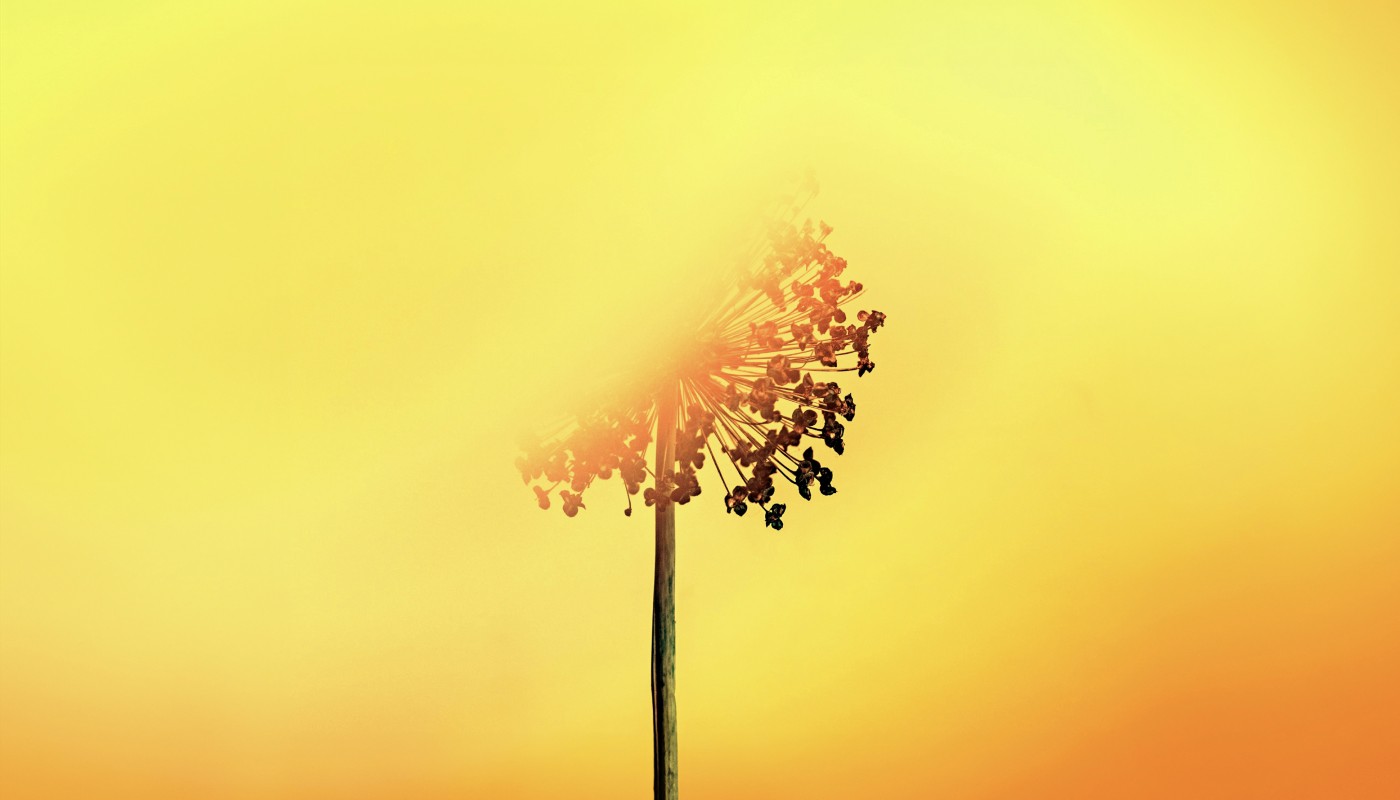
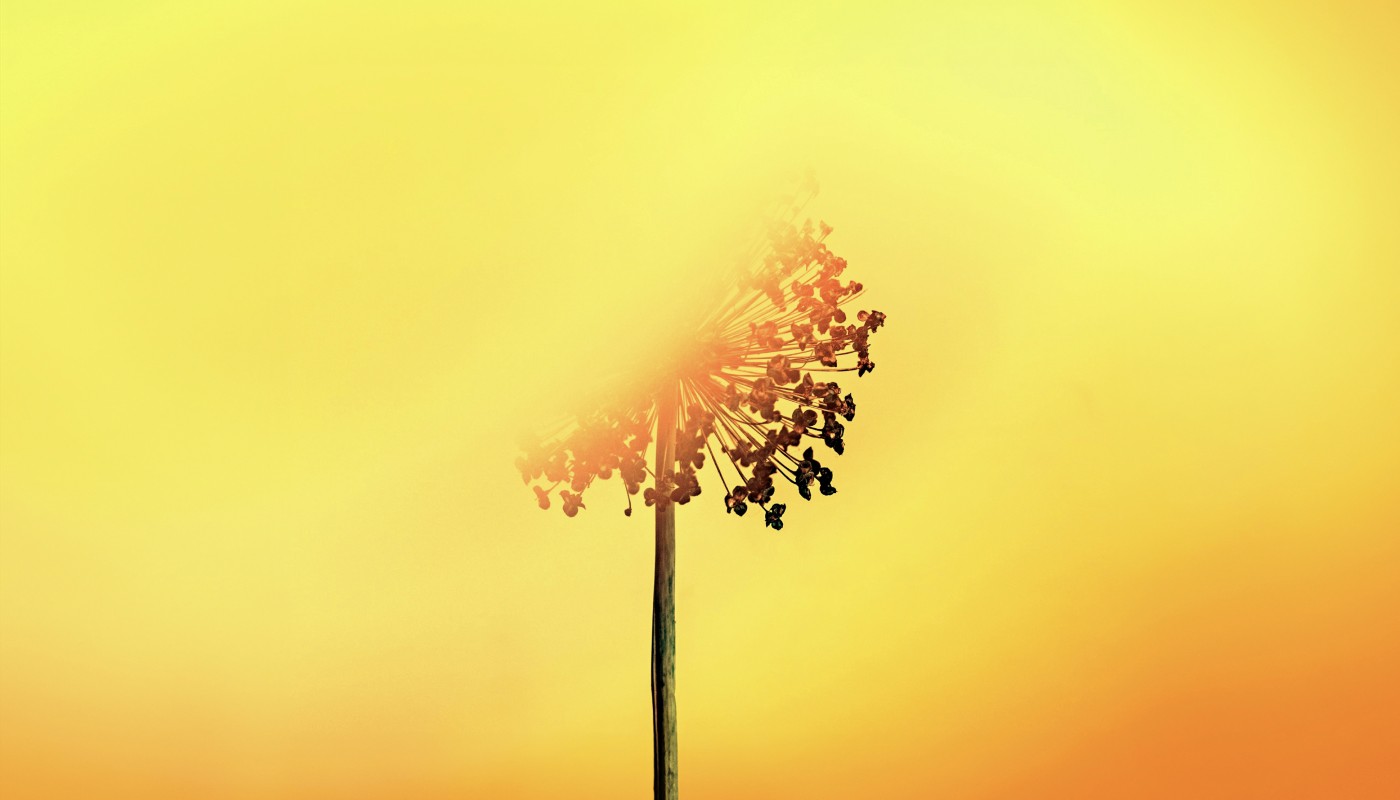
NIST’s New Quantum Cryptography Standards: What You Need to Know
Apr 25, 2025
Read our latest commentary and research on the post-quantum encryption space
Read our latest commentary and research on the post-quantum encryption space


Cracking RSA with Fewer Qubits: What Google's New Quantum Factoring Estimate Means for Cybersecurity


Quantum Arms Race: U.S. Defense Intelligence Flags Rivals’ Growing Military Use of Quantum Tech


Quantum Threats and Bitcoin: Why BlackRock’s Warning Matters for the Future of Crypto Security


Sudbury's SNOLAB Ventures into Quantum Computing Research


Lockheed Martin and IBM Pioneer Quantum-Classical Hybrid Computing for Complex Molecular Simulations


Why the Moon Matters for Quantum Computing: From Helium-3 to Off-Planet Quantum Networks


NIST Approves Three Post-Quantum Cryptography Standards: A Milestone for Digital Security


Scientists Connect Quantum Processors via Fiber Optic Cable for the First Time


Quantum Computing and Encryption Breakthroughs in 2025: A New Era of Innovation


How CISOs Can Defend Against the “Harvest Now, Decrypt Later” Threat


NVIDIA Expands Quantum and AI Ecosystem in Taiwan Through Strategic Partnerships and Supercomputing Initiatives


Quantum Annealing Breakthrough: Quantum Computer Outperforms Fastest Supercomputers

Cracking RSA with Fewer Qubits: What Google's New Quantum Factoring Estimate Means for Cybersecurity

Quantum Arms Race: U.S. Defense Intelligence Flags Rivals’ Growing Military Use of Quantum Tech

Quantum Threats and Bitcoin: Why BlackRock’s Warning Matters for the Future of Crypto Security

Sudbury's SNOLAB Ventures into Quantum Computing Research

Lockheed Martin and IBM Pioneer Quantum-Classical Hybrid Computing for Complex Molecular Simulations

Why the Moon Matters for Quantum Computing: From Helium-3 to Off-Planet Quantum Networks

NIST Approves Three Post-Quantum Cryptography Standards: A Milestone for Digital Security

Scientists Connect Quantum Processors via Fiber Optic Cable for the First Time

Quantum Computing and Encryption Breakthroughs in 2025: A New Era of Innovation

How CISOs Can Defend Against the “Harvest Now, Decrypt Later” Threat

NVIDIA Expands Quantum and AI Ecosystem in Taiwan Through Strategic Partnerships and Supercomputing Initiatives

Quantum Annealing Breakthrough: Quantum Computer Outperforms Fastest Supercomputers

Quantum Computing's New Frontier: How the $1.4 Trillion US–UAE Investment Deal is Shaping the Industry

Quantum Computing Meets Cancer Research: A New Frontier in Drug Discovery

Quantum Industry Leaders Urge Congress to Reauthorize and Expand National Quantum Initiative
Groundbreaking Progress in Quantum Gate Fidelity
In a landmark advancement for quantum computing, scientists at QuTech—a collaboration between Delft University of Technology and the Netherlands Organisation for Applied Scientific Research (TNO)—have shattered previous records by developing ultra-precise quantum gates on a diamond-based chip. Their system achieves error rates as low as 0.001%, a critical milestone that brings fault-tolerant quantum computing closer to reality.
This breakthrough, published in Physical Review Applied, demonstrates that diamond spin qubits can perform high-fidelity quantum operations, overcoming one of the field’s most persistent challenges: maintaining precision across long sequences of computations.
Why Quantum Gates Must Be Nearly Perfect
Quantum computers rely on quantum gates—the fundamental operations that manipulate qubits—to perform calculations. Unlike classical bits, qubits are highly sensitive to noise, meaning even tiny errors can cascade and derail computations. For quantum error correction (QEC) to work effectively, gate errors must remain below 0.1%, a threshold known as the "fault-tolerant limit."
Until now, most quantum platforms struggled to consistently meet this benchmark. QuTech’s diamond-based system not only surpasses it but does so with some gates operating at 0.001% error rates, making it one of the most reliable quantum technologies to date.
Diamond Qubits: A Leading Candidate for Scalable Quantum Computing
The researchers utilized nitrogen-vacancy (NV) centers in diamond—atomic-scale defects where a nitrogen atom replaces a carbon atom, trapping an extra electron. These defects act as natural qubits, offering several advantages:
1. High-Temperature Operation
Unlike superconducting qubits, which require near-absolute-zero temperatures, diamond spin qubits can function at up to 10 Kelvin, significantly reducing cooling demands.
2. Exceptional Noise Resistance
The diamond lattice naturally shields qubits from electromagnetic interference, making them more stable than competing technologies.
3. Built-in Photon Coupling
NV centers can interact with light, enabling quantum networking—a crucial feature for future distributed quantum computing.
Despite these benefits, previous attempts to build high-fidelity quantum gates with diamond qubits were hampered by material imperfections and control challenges.
How QuTech Achieved Near-Perfect Quantum Gates
The team’s success hinged on three key innovations:
1. Ultra-Pure Diamond Crystals
Natural diamonds contain carbon-13 isotopes, which introduce magnetic noise. The researchers used isotopically purified diamonds, drastically reducing decoherence.
2. Advanced Gate Design
By carefully engineering microwave and laser pulses, they minimized unwanted interactions between qubits and their environment.
3. Precision Characterization with Gate Set Tomography
Instead of traditional benchmarking, the team used gate set tomography (GST), a method that provides a complete quantum description of each gate. "GST was essential—it revealed hidden errors that standard tests miss," explains co-author Jiwon Yun.
Stress-Testing the Quantum Gates
To validate their system, the researchers ran an 800-gate quantum algorithm, far exceeding typical test sequences. The results matched theoretical predictions with near-perfect accuracy, proving the gates’ reliability.
"This level of precision means we can trust our quantum operations even in long computations," says lead author Hans Bartling.
The Next Challenge: Scaling Up
While this two-qubit demonstration is a major leap, building a practical quantum computer requires thousands—or even millions—of qubits. Key hurdles include:
Maintaining low error rates as qubit count increases
Integrating diamond qubits with photonic interconnects
Developing scalable fabrication techniques
QuTech is tackling these challenges through its collaboration with Fujitsu, combining expertise in quantum hardware, control electronics, and system architecture.
"We’re not just improving qubits—we’re rethinking the entire quantum computing stack," says Tim Taminiau, the project’s lead scientist. "The next phase will require industry partnerships to transition from lab experiments to real-world applications."
Implications for the Future of Quantum Computing
This breakthrough has far-reaching implications:
✔ Fault-Tolerant Quantum Computing – Proves diamond qubits can meet the strict error thresholds needed for QEC.
✔ Hybrid Quantum Systems – NV centers could link superconducting or trapped-ion qubits via photons.
✔ Quantum Networks – Enables long-distance entanglement for secure communication.
Conclusion: A Major Step Toward Practical Quantum Computers
QuTech’s diamond-based quantum gates represent a turning point in the quest for scalable quantum computing. While challenges remain, this work demonstrates that high-fidelity quantum operations are achievable, paving the way for error-corrected, large-scale quantum processors.
As research progresses, diamond qubits may soon compete with—or even surpass—other leading quantum technologies, bringing us closer to solving problems beyond the reach of classical supercomputers.
Reference:
H.P. Bartling et al., "Universal high-fidelity quantum gates for spin qubits in diamond," Physical Review Applied (2025).
DOI: 10.1103/PhysRevApplied.23.034052
Let's talk!
Office:
1535 Broadway
New York, NY 10036
USA
Local time:
20:13:00
Let's talk!
Office:
1535 Broadway
New York, NY 10036
USA
Local time:
20:13:00